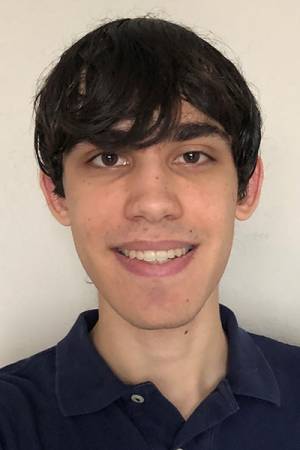
Abstract:
Climbing robots can operate in steep and unstructured environments that are inaccessible to other ground robots, with applications ranging from the inspection of artificial structures on Earth to the exploration of natural terrain features throughout the solar system. Climbing robots for planetary exploration face many challenges to deployment, including mass restrictions, irregular surface features, and communication delays. We propose a hierarchical approach to overcome these obstacles via underactuated design, internal force control, and autonomous path planning.
We apply this framework to the design of a novel quadrupedal rock-climbing robot for planetary exploration. We simplify the microspine manufacturing process via 3D printing, facilitating rapid prototyping of new designs. Fully passive grippers enable secure grasping on irregular terrain while avoiding the mass, complexity, and slow speed of previous actuated solutions. An optimization-based force control strategy generates internal forces between the grippers to further improve adhesion. We also present a magnetic-wheeled robot for the inspection of steel structures. The robot relies on a passive suspension and multi-functional mechanisms for obstacle traversal, maneuverability, sensor deployment, and transitions across interior corners.
Finally, we propose a path planning approach for navigating across arbitrary surface geometries represented by point clouds. The algorithm will evaluate the robot’s stability at each key frame along a path, including transitions between contact modes, to ensure the safety of the solution. By combining all three levels of this hierarchy we aim to enable robust and autonomous climbing in a wide range of steep and unstructured environments.
Thesis Committee Members:
Aaron M. Johnson, Chair
William (Red) Whittaker
Zeynep Temel
Spencer Backus, NASA Jet Propulsion Laboratory