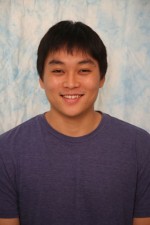
10:30 am to 12:00 pm
NSH 3305
Seungmoon Song
Ph.D. Thesis Defense
Abstract:
The neural control of human locomotion is not fully understood. As current experimental techniques provide only partial and indirect access to the neural control network, our understanding remains fragmentary with large gaps between detectable neural circuits and measurable behavioral data. Neuromechanical simulation studies can help bridging these gaps. By testing a hypothesized controller in neuromechanical simulations, one can evaluate the plausibility of the controller and propose experimental studies which can further investigate the hypothesis. Better understanding the control of human locomotion will change the way we design rehabilitation treatment and engineer assistive devices.
This thesis first investigates how much of human locomotion control can be explained by spinal reflexes using neuromechanical simulations. It is known that the spinal control is essential in generating locomotion behaviors in humans, which leads to two central questions: “how does the lower layer controller in the spinal cord generate the motor patterns?” and “how is this lower layer controller modulated by the higher layer brain control to realize different locomotion tasks?” To investigate these questions, we propose a hierarchical control model with two layers, where the lower-layer control consists of spinal reflexes, and the higher-layer sends a few commands to modulate this lower layer control. In neuromechanical simulations, this model can generate diverse human locomotion behaviors, including walking and running, adapting to slopes and stairs, and changing locomotion directions and speeds. Furthermore, its reactions to a range of unexpected disturbances during normal walking are remarkably similar to those observed in human experiments. The simulation results suggest following answers to the central questions: “the motor patterns of many human locomotion behaviors can be generated by chains of reflexes” and “different locomotion behaviors can be realized by a reflex-based unified controller that is modulated by the higher-layer control.”
The latter part of this thesis presents three studies of using the neuromechanical control model either as a simulation testbed for studying human locomotion or as a robotic controller for legged machines. First, the neuromechanical model is used to study human foot biomechanics. The walking simulations with different foot designs suggest that the windlass mechanism in human feet saves metabolic cost during walking, and this saving does not come from the compliance of the feet, which is one component of this mechanism. Second, the age-related skeletal, muscular, and neural changes are applied to the model to investigate why the metabolic cost increases and the regular walking speed reduces in elderly people. The increase of metabolic cost of the elderly model is mostly attributed to weakened muscles, and we find muscle fatigue as a plausible performance criterion that suggests slower walking speed for the elderly model. In the last study, we adapt the neuromechanical model for a bipedal robot ATRIAS. With the controller, ATRIAS could walk on a rough terrain with unknown height changes of ± 20 cm in a sagittal plane physics simulation.
Thesis Committee Members:
Hartmut Geyer, Chair
Christopher G. Atkeson
Stelian Coros
Auke J. Ijspeert, EPFL